In 2007, David Smith, a doctor of internal medicine and founder of a company that makes wound dressings, gave a presentation at a medical conference in Maryland. Afterward an audience member, worried by mounting reports of traumatic brain injury from blasts among American soldiers, mentioned, of all things, woodpeckers. If someone could figure out how woodpeckers do it—they slam their beaks into trees thousands of times per day, generating forces far beyond what most people experience in car wrecks—then maybe we could better protect soldiers.
The question sent Smith’s own head spinning. “I knew instantaneously that this was something woefully overlooked,” Smith said. Nature was full of animals that routinely generated massive forces on their brains without apparent damage. They included bighorn sheep; whales that ram each other; and birds that dive headlong, at 500 feet per second, into the water. How did the animals avoid brain injury?
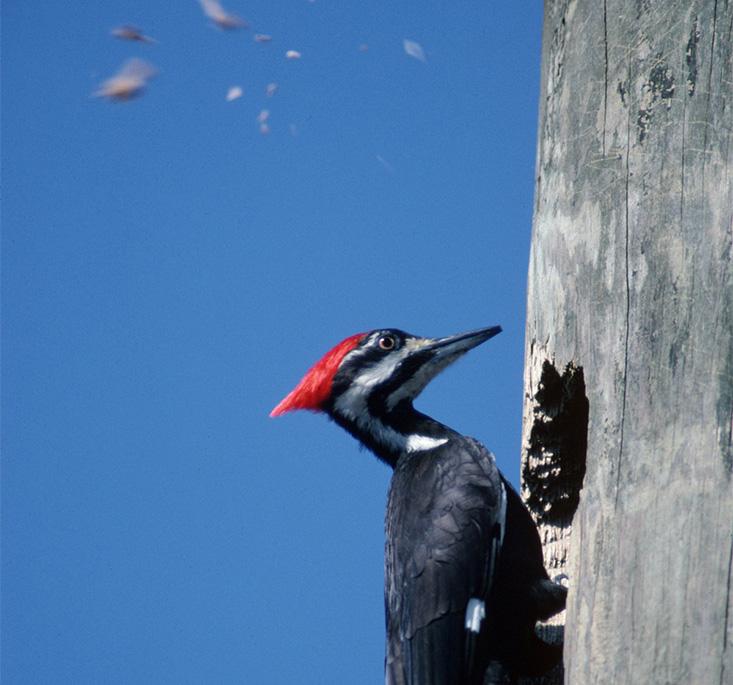
The animals’ secrets wouldn’t apply just to soldiers, Smith knew. Reports were accumulating of a dementia-like syndrome that afflicted former football players, called chronic traumatic encephalopathy. The condition, scientists thought, wasn’t necessarily caused by full-blown concussions—the kind that knock you out—but by repeated lighter blows over a long period of time.
The problem is the human head isn’t “designed” to take repeated hits. The brain floats in fluid slightly removed from the skull. But because it’s not the same density as the fluid around it—and even the different tissues within the brain aren’t of equal density—when you collide with another player, the brain moves through the surrounding fluid, smashing and deforming against the skull. That slosh, or “rattle,” as some call it, compresses and shears neurons, injuring them. Something called cavitation may also occur: the formation of gas bubbles driven by a rapid change in fluid pressure. When you’re hit on the football field, in other words, parts of your brain may fizz like a just-opened can of soda.
Smith had an eclectic background: He had an M.D., math skills, and a degree in chemistry. His company’s wound-care products accelerated healing by maintaining moisture at the wound site. He also loved football. He played in high school, and swam competitively. So he dove into the literature on woodpecker physiology, trying to discern how the animal protected itself.
He immediately fixated on an unusual feature of the woodpecker’s physiology: its long tongue, which wraps underneath and up and around the back of its skull. A muscle that rests against the animal’s jugular veins moves the tongue. So the woodpecker could, in theory, use its omohyoid muscle, as it’s called, to constrict these veins, reducing the outflow of blood from its skull. This was, Smith thought, the woodpecker’s secret. It likely clinched its jugular vein with its long omohyoid muscle, protecting against brain slosh by filling its brain with blood.
Smith wondered how he could replicate this in people. One possibility was to elevate carbon dioxide. We produce CO during regular cellular processes, and expel it when we exhale. But if blood CO goes too high, indicating an oxygen-poor environment, blood flow to the brain increases to ensure that the all-important organ has sufficient oxygen. That meant one way to swell the brain was to increase blood CO.
When you’re hit on the football field, your brain may fizz like a just-opened can of soda.
In fact, Smith suspected that woodpeckers and many other birds did this as well—they could deliberately elevate blood CO. Birds have a unique respiratory system which, in addition to lungs, features air sacs distributed throughout their bodies. The sacs are connected via birds’ hollow, “pneumatic” bones. Smith thought that maybe this extended respiratory system allowed some birds to “re-breathe”—to re-inhale off-gassed CO—increasing carbon dioxide on demand, boosting the blood volume of their brains, and protecting their brains during the many collisions of avian life.
Smith needed evidence this might apply in people. So he teamed up with Gregory Myer, director of research in sports medicine at Cincinnati Children’s Hospital Medical Center. (Today Smith is a visiting research scientist at the center.) The CO hypothesis afforded a prediction. High altitude changes the ratio of carbon dioxide to oxygen in the blood, producing a slight swelling of the brain. (At really high altitudes, the swelling can lead to the dizziness and vomiting of altitude sickness.) Would this mild, altitude-induced “edema” protect people from head injury?
Myer and his colleagues looked at a database of NFL games played around the country. Games played above 644 feet, they found, reported 30 percent fewer concussions than games played at lower altitudes. The thinner air—or perhaps the body’s adaptive response to lower oxygen—seemed to protect players’ brains.
Smith has a knack for getting scientists on board with his ideas even if, at first blush, they’re not sure if he’s crazy or a genius. Ultimately it was another research scientist named Joseph Fisher who devised a more practical way to mimic the woodpecker. Fisher, a professor at the University of Toronto, studies how gases diffuse in the blood, particularly carbon dioxide. He agreed to hear out Smith, formaldehyde-soaked woodpecker in tow, over dinner. “He had no data, but he had a brilliant insight,” Fisher said.
Fisher didn’t think Smith’s initial approach was right, though. He wanted to raise people’s CO artificially, induce mild brain swelling, and protect against trauma that way. But high blood CO would be uncomfortable, Fisher thought, leaving people feeling as if they were suffocating and gasping for air.
Fisher, an anesthesiologist by training, thought a second approach, one more in line with the woodpecker’s omohyoid solution, made more sense. He knew from personal experience that just a little pressure on a person’s neck would make the brain swell. He’d seen it happen. Brain surgeons sometimes complained that the brain swelled up during surgery, so they dispatched Fisher to find out what was crimping their patients’ necks. (The problem might be that the patient’s head was slightly turned, applying pressure to the jugular veins.)
So forget CO, Fisher thought. All you needed was to press lightly on the neck. Fisher bought a pair of headphones at an electronics store, bent the metal band a little, and placed them around his neck with the pads against his jugular veins. He felt his face flush. An avid cyclist, Fisher would wear that jerry-rigged collar for the next two years while biking.
“Our brain already has a helmet, it’s called a skull. Why put another shell on the skull?”
Not everyone agrees with Smith’s basic scientific premise. Andrew Farke, a scientist at the Raymond M. Alf Museum of Paleontology in Claremont, California, who studies head-butting animals, said no one has looked into the question of whether woodpeckers and rams really bang their heads without hurting their brains. It’s widely assumed these animals don’t have problems from a lifetime of head-smashing, he noted, but it’s not proven. Maybe woodpeckers or rams don’t live long enough in the wild to develop degenerative conditions like CTE. “The stereotype that they’re not suffering any ill consequences is probably something that should be evaluated,” he said.
And about the CO hypothesis. Smith thinks that bighorn sheep, which slam into each other at 40 miles per hour, generating up to 500 Gs of force, “rebreathe” through their hollow horns, raise their blood CO, increase the blood volume in heads, and protect their brains during those fearsome collisions. But the animals’ hollow horns are nearly impossible to breathe through, Farke said. The purpose of the hollows, he said, is to create distance between the area of impact and the brain. And the horns themselves help diffuse force. They’re sheathed in the same stuff that hair is made of—keratin—and they flex slightly during collisions, “like a crumple zone on a car,” Farke said.
Farke added that woodpeckers’ unique wrap-around tongues can press on their jugulars. But the birds also have many other adaptations, including shock-absorbing areas of the beak and body. “It’s very rarely a single magic bullet,” Farke said. “As with pretty much everything, it’s a whole bunch of adaptations.”
And yet a rodent study conducted by Julian Bailes, a concussion expert and chairman of the department of neurosurgery at the North Shore University Health System in Evanston, Illinois, stunned everyone with its results. Rats that wore a collar-like apparatus around their necks that lightly compressed their jugular veins while they were knocked on the head saw an 83 percent reduction in brain damage compared to rats that didn’t. “I was dumbfounded,” Fisher said of the results. “I would have been happy to have 10 percent improvement.”
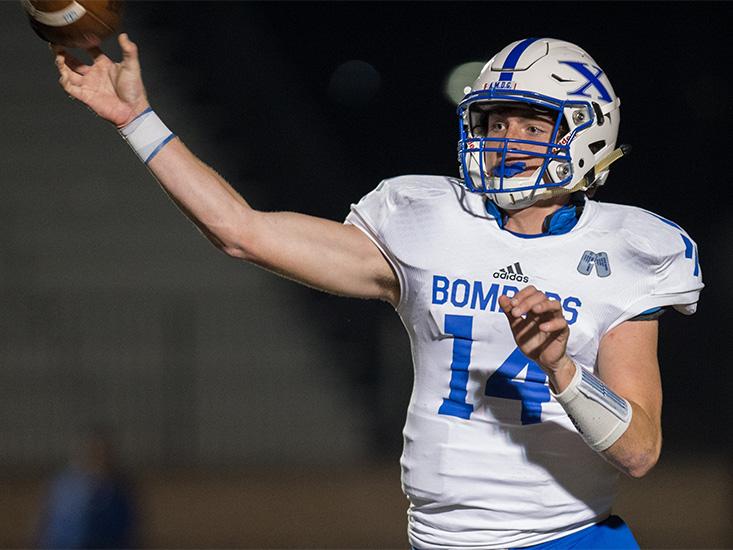
Football helmets were originally designed to prevent skull fractures, which they do fairly well. But to preclude movement inside the skull, helmets would have to significantly slow down the period of impact. A helmet able to achieve this feat might look like a giant, fluffy marshmallow—unwieldy, and perhaps ergonomically impossible to wear. Myer, for one, thinks that a helmet, no matter how well designed, is unlikely to prevent CTE in sports like football. “Our brain already has a helmet, it’s called a skull,” he said. “Why put another shell on the skull? You’re just adding mass.”
Smith enlisted a sportswear company now called Q30 Innovations to design a collar for human use. The device they’ve come up with is made of light, springy metal coated in a plastic polymer. It’s C-shaped, slides over the back of the neck, and leaves the windpipe untouched. The collar increases blood in the brain by about a teaspoon. That’s equivalent to the extra blood that pools in your head when you lie down. Or when you yawn. “If you think this is dangerous physiology, for God’s sake, don’t yawn,” Smith said.
To test the collar in people, the scientists couldn’t very well knock people repeatedly in the head deliberately. So Myer turned to two high school football teams in Cincinnati. The players agreed to have an array of sensors placed in their helmets, allowing the scientists to see, after games, how many collisions the players had experienced, and at what force. In one game, Elijah Payne, a 225-pound middle linebacker at St. Xavier High School, registered 31 hits, some of them 20 times the force of gravity.
The collar took some getting used to, Payne said, particularly on hot days during intense practice. He could feel the pressure on his neck. Once when he was running sprints, he almost took it off. “I’d be lying if I said it wasn’t tough,” he said. But Payne persevered, and with time he stopped noticing the collar at all. By the end of the season, it had become just another piece of football equipment.
Before and after the season, Payne and his classmates underwent a type of brain scan called diffusion tensor imaging, which measures changes in the brain indicative of damage. By season’s end, each player had experienced, on average, about 800 head impacts greater than 20 Gs. And when Myer compared scans from before and after, he noted changes in the brains of kids who hadn’t worn the collar—evidence of damage. The kids who had worn the collar, on the other hand, saw significantly fewer changes. Their brains hadn’t suffered the same way. The findings were also replicated in hockey players. What worked for woodpeckers seemed to work for humans. A little extra blood in the skull swaddled the brain enough to reduce damage.
The studies have some weaknesses. There’s no placebo group, no players wearing a collar that does nothing—something that’s hard to do in these kinds of studies, Myer said. And no one knows if the reduced damage seen in the collar-wearing group, as measured by diffuse tensor imaging, will translate to a lower risk of CTE later. So far, CTE can only be diagnosed after death.
Still, what began years ago as one man’s obsession with woodpeckers may soon yield a commercial product that protects your brain by gently squeezing your neck. Q30 Innovations, and another company, Performance Sports Group, are trying to gain FDA approval to sell the collar as a medical device. Smith, who’s 57 and owns a patent for the collar, is about to leave medicine to focus on a line of research that began with an obsessive foray into woodpecker physiology. “I really feared for the future of football,” he said. “I actually don’t anymore. I think we can prevent CTE.”
Moises Velasquez-Manoff is a journalist and author of An Epidemic of Absence: A New Way of Understanding Allergies and Autoimmune Diseases.
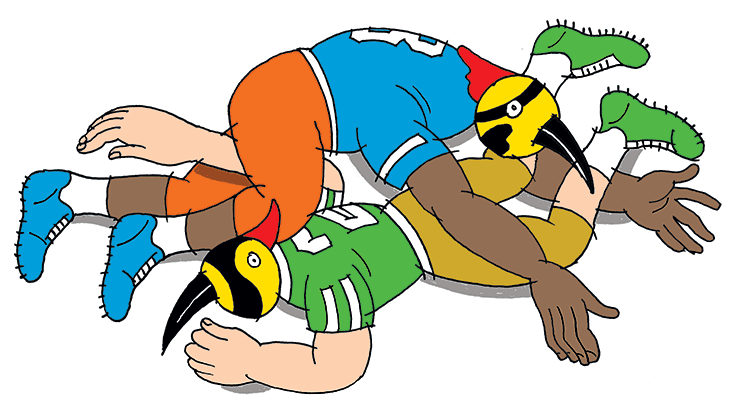