I arrived on the second day of creation. Laurie Barge had invited me to spend the day in her lab, modeling the origin of life. She is a research scientist at NASA’s Jet Propulsion Laboratory in Pasadena and, with her colleague, the pioneering geologist Michael J. Russell, a member of the NASA Astrobiology Institute. The task was to make a miniature hydrothermal vent under conditions that simulated the primeval ocean, 4 billion years ago. Such vents are at the heart of a scientific creation story so counterintuitive it could hardly be true, yet so logical that in broad strokes it almost must be.
On the first day, Barge and her students had created the oceans. They started with distilled water and bubbled nitrogen through it to displace oxygen gas, which had not been present on the early Earth. We suspended two early Earths on steel stands in lab beakers inside a fume hood. Into the oceans, we added iron chloride, which turned the water the color of flat beer. I inserted a pipette tip into the bottom of each vessel and pumped in sodium sulfide to simulate hot fluid rising through breaks in the Earth’s crust. The sodium recombined with chloride to make saltwater, while the sulfur paired off with iron to form iron sulfide, which precipitated out of the solution and accreted into hollow chimneys. Similar chimneys formed at hydrothermal vents in the late Hadean eon 4 billion years ago, and they form still, from the abyssal seas to Barge’s laboratory.
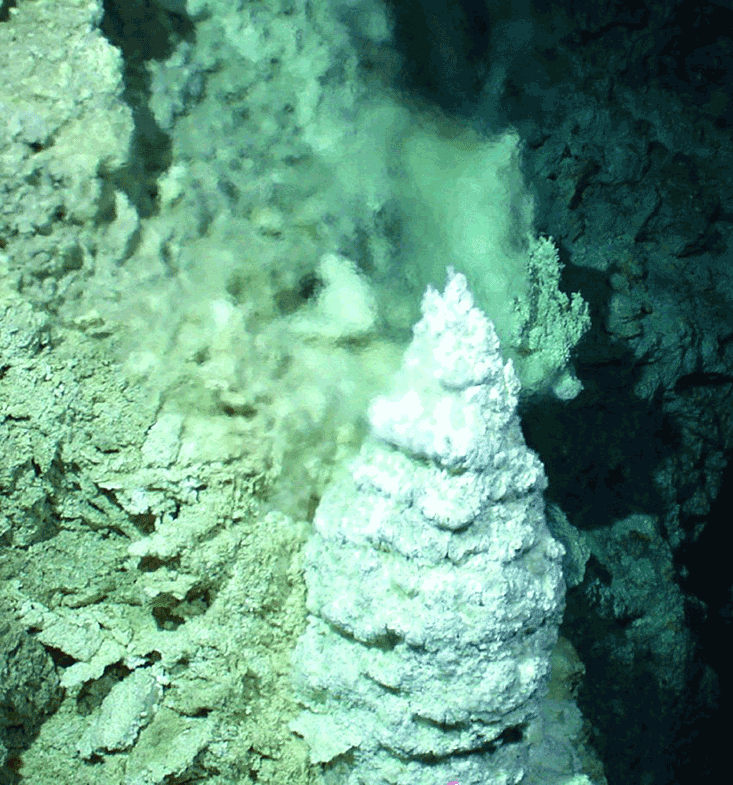
The idea that life began at hydrothermal vents challenges the older, familiar scientific creation story of the “primordial soup.” In a letter to Joseph Hooker in 1871, Charles Darwin fancied that life originated “in some warm little pond, with all sorts of ammonia and phosphoric salts, light, heat, electricity etc., present,” in which a “protein compound was chemically formed ready to undergo still more complex changes.” In the 1920s, the Russian scientist Aleksandr Oparin and the British polymath J.B.S. Haldane independently fleshed out models of how life might have arisen this way, out of an ancient ocean with the consistency, Haldane said, of a “hot, dilute soup.” The name stuck.
A little bit of that ancient Lost City lies within every cell.
In 1953, Stanley Miller, a graduate student of the Nobel laureate chemist Harold Urey, succeeded in making soup in the lab. He set up flasks with presumptive primordial ocean and atmosphere, sparked the system with artificial lightning, and collected the compounds produced. He found many tantalizing ones, including several amino acids. Miller-Urey chemistry has become a shorthand in origin-of-life research, while Miller’s numerous students have themselves gone forth and multiplied. In origin-of-life research today, “the soup” connotes models where life begins on or near the ocean surface via Miller-Urey chemistry, with lightning or another energy source repeatedly jolting molecules into states of ever-greater complexity, until Darwinian evolution takes over.1
The soup has an intuitive appeal: From it you can derive the building blocks of life. But it also has a fatal flaw: No matter what it produces, it’s dead. Lightning bolts may spark biochemical reactions, but the energy quickly dissipates and the system returns to equilibrium. Primordial soup requires evolution to go uphill, thermodynamically, toward increasing order. It’s like one of those “gravity hills” much documented on the Internet, where cars seem to roll uphill. Starting from heat, rocks, and seawater, amino acids and nucleotides self-assembled. They organized themselves into even more ordered molecules such as enzymes and proteins. From them evolution built the first cells, and eventually redwoods and roses, honeybees and apple trees, hyenas and humans.
But the gravity hills are a trick of perspective. A carpenter’s level would reveal the truth, but you don’t often see one in the “like-magic” gravity hill videos. The laws of physics, in fact, remain intact. So too, say the vent theorists, with the emergence of life.2-5 Evolution only seems to move toward greater order; in the larger scheme, it’s downhill all the way. Vent models posit that given the initial conditions, the emergence of life was not a near-miracle. It was inevitable.
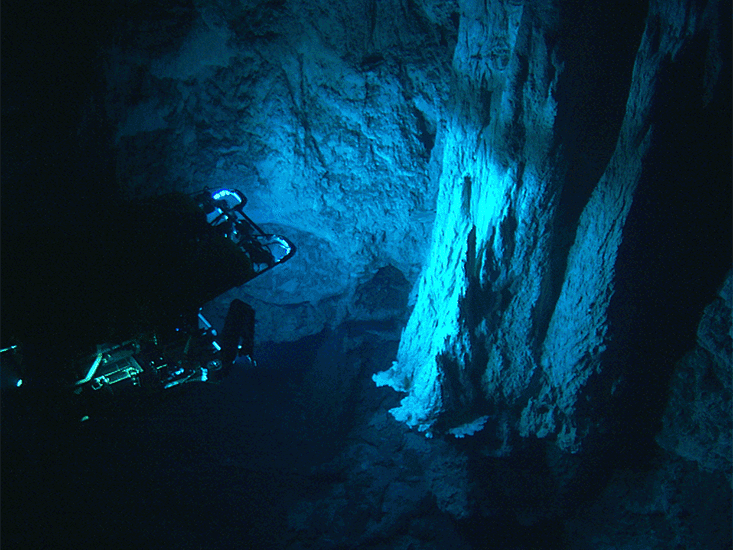
Hydrothermal springs were discovered by oceanographers in 1977 at the Galápagos Rift, in the East Pacific.6 Then, in 1979 on the crest of the East Pacific Rise at 21 degrees North, great chimneys were discovered spewing sooty, super-hot acid into the cold, lightless depths. Accurately if not poetically, the structures became known as “black smokers.” Researchers were stunned to find the areas around black smokers to be teeming with life, from fish to countless new species of microbes. In 1981, Jack Corliss, one of the oceanographers on the Galápagos Rift expedition, together with microbiologists John Baross and Sarah Hoffman, suggested that submarine hot springs “provide all of the conditions necessary for the creation of life on Earth.” You didn’t need light or lightning. There was no soup.
The Millerites returned fire. The vents were far too hot to support life, wrote Miller and his erstwhile student Jeffrey Bada. Amino acids and nucleic acids, if formed, would break down almost instantly. Sugars would melt. Life could not possibly start in such a hostile environment. If anything, they wrote, “the vents would have been important in the destruction rather than in the synthesis of organic compounds in the primitive oceans.”7
A lush green Earth teeming with life pulls more heat out of the hot center of the planet and out of the sun than does, say, Mars.
Russell and his colleague Allan Hall, now an archaeologist at the University of Glasgow, chimed in. You’re right, they told the Millerites: Black smokers are too hot, and acidic, for life to have formed there. Near them, though, they wrote, one ought to find mineral tubes that emit lukewarm, alkaline fluid. They would be ideal sites for the emergence of life.8
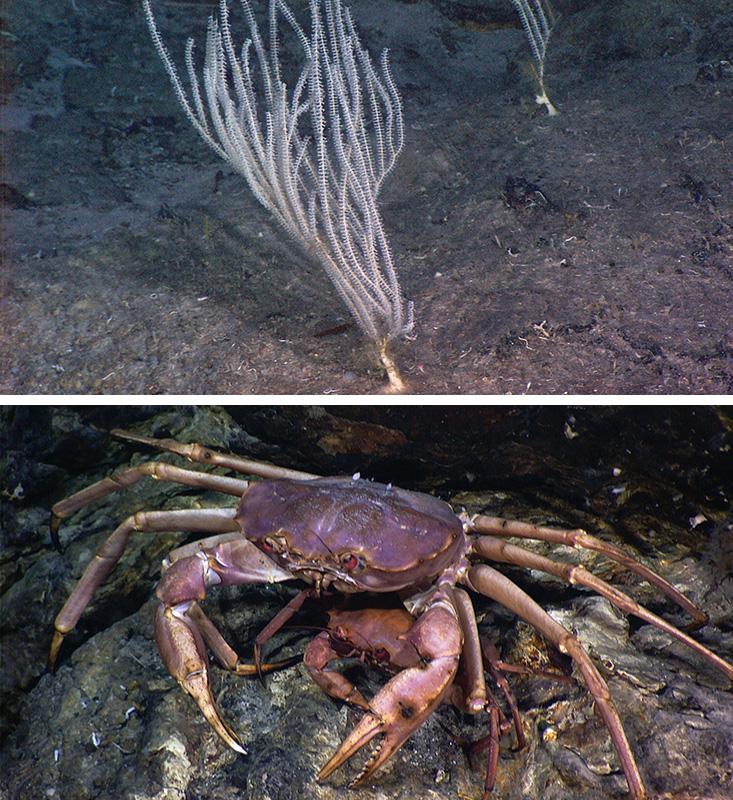
On Dec. 4, 2000, those tubes were found, just as Russell had predicted, though quite by accident. A research team led by Scripps Institution of Oceanography’s Donna Blackman, Deborah Kelley of the University of Washington, and Duke University’s Jeffrey Karson was exploring the Atlantis Massif, a 10-mile long dome named after the mythical island city that, according to Plato, sunk into the North Atlantic after being defeated by Athens. Near the end of the month-long expedition, the robotic submersible strayed off its prearranged course, following some fish that seemed to be mugging for the camera. Suddenly, researchers saw a vast system of pearly white structures loom up on the remote monitor: cones, spires, and frosted windowpanes, some the size of buildings. Irresistibly, they named the site “Lost City.”9
Exploration revealed that the towers of Lost City emitted a clear, warm, alkaline fluid into the slightly acidic ocean. The margins separated warm from cool, concentrated from dilute, lower pH from higher pH. An early Earth “Lost City” would have developed in a carbonated, and thereby acidic ocean. Its porous walls, made of iron sulfides and oxides, would have made that precursor Lost City a weak but vast battery.10 Living cells, too, are surrounded by a membrane that separates an alkaline interior from a slightly acidic exterior. “The Last Universal Common Ancestor” of life, Barge says, “was functioning off of electron and proton gradients, like life does today.” Whether animal, plant, fungus, or bacterium, all organisms recapitulate the chemistry of oxidation and reduction found in the warm alkaline vents. A little bit of that ancient Lost City lies within every cell.
As Barge and I watched the experiment, the iron-sulfide chimneys began to form elaborate structures. The rising fluid built its own exhaust pipe. A bit of crystal blocked the flow; the fluid found a new path as it rose; the structure branched. The result was startlingly plant-like. Premodern alchemists made similar “chymical gardens,” and a nearly forgotten 19th-century biologist named Stéphane Leduc believed that these organic forms reflected the principles of biological growth. “The chain of life,” Leduc said, is “a continuous one, from the mineral at one end to the most complicated organism at the other.”
Modern hydrothermal vent models suggest an explanation of how that chain was built. The giant battery of an early Lost City drives an engine that makes complex molecules, mainly out of carbon, hydrogen, and oxygen. Iron sulfide, as well as other small molecules found at the vents, act as “coenzymes”—catalytic nanoengines that drive the reactions lying at the heart of all metabolism. The chimneys, in short, have a kind of metabolism, which takes energy from hydrogen, CO, and other molecules, and uses it to build more complex molecules, mainly out of carbon, hydrogen, and oxygen. The most ancient metabolic pathways in biology recapitulate the chemistry of that early Lost City.
Neither art, nor war, nor NASCAR, nor smart phones were inevitable, but all can be seen as work done by the human entropy engine.
What most goes against our intuition is that complex structures can be better dissipaters of energy than simpler ones.11 Catalysts help you up an energy hill so that you can drop even further down on the other side. Casting our gaze across the entirety of biological evolution, each organism is such an energy hill. It forms only if it is thermodynamically favored—if by pumping energy uphill to create it, even more energy is released. A lizard, for example, requires more energy to make than a lizard’s-worth of E. coli, but it consumes more energy at a greater rate. A world that contains both lizards and bacteria, then, is energetically favored over a world of only bacteria. A world that also includes warm-blooded cows, munching grass and emitting heat, methane, and fertilizer, is an even better entropy engine; one with tigers is better still. It is the ecosystem that is energetically favored: A lush green Earth teeming with life pulls more heat out of the hot center of the planet and out of the sun, releasing it into cold, dark space, than does, say, Mars. Our biosphere is but a sophisticated icepack for the sun.
A giant dissipation engine came on the scene with Homo sapiens. The history of technology is ultimately one of developing ever more powerful methods of extracting energy from the earth and sun: fire, cooking, agriculture, mining, smelting, logging, steam engines. Like a trickle of water flowing downhill, the exact path of neither evolution nor culture is predetermined—only the overall trend. Thus, neither art, nor war, nor NASCAR, nor smart phones were inevitable, but all can be seen as work done by the human entropy engine. In this light, our dissipative tendencies are not an aberration, but thermodynamically required.
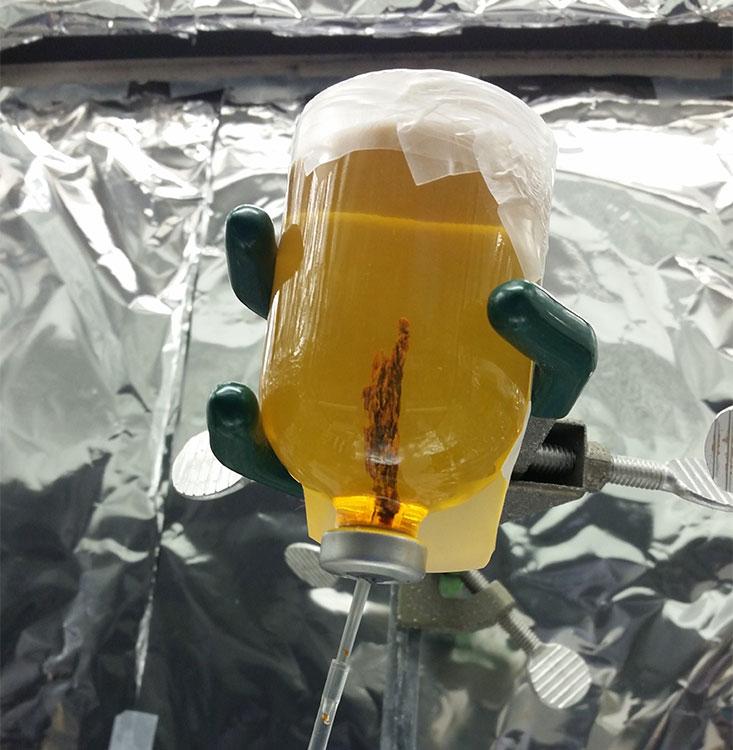
If Barge and I watched our laboratory model long enough, would it too evolve a metabolic pathway? Enzymes? Genes? Barge is taking the first steps in that direction, though the steps are small. Instead of building a chimney, she deposits iron sulfide and other minerals onto a porous, inert disk. That disk can serve as a membrane between, say, a positively charged fluid and a negatively charged one. Barge measures the voltage and pH differences across the membrane—the flow of electrons and protons. Those currents drive chemical reactions that are fundamental to life. The next step will be to get the chemical reactions to evolve more complex molecules. “You could also set up experiments,” she says, “to test the emerging feedback of organics with minerals.” Simple catalysts could favor reactions whose products were more complex catalysts, which could produce yet more complex catalysts, in a feedback loop leading ultimately—way down the road—to protein and DNA.
In one of my two worlds, the chimney developed a slender stalk, and then a heavy bulb swelled at the tip. “That one will probably break,” Barge says. It did: a dead end of evolution. But the chimney in the other flask grew a fat, flat base and tapered mountainously into a series of peaks that, if you were a water flea, would seem majestic. And Laurie examined it and she said that it was good.
Nathaniel Comfort is the Baruch Blumberg Chair in Astrobiology at the Library of Congress/NASA and a professor of the history of medicine at Johns Hopkins University. His most recent book is The Science of Human Perfection. He tweets from @nccomfort.
References
1. Plaxco, K.W. & Gross, M. Astrobiology: A Brief Introduction Johns Hopkins University Press, Baltimore, MD (2006).
2. Martin, W. & Russell, M.J. On the origins of cells: A hypothesis for the evolutionary transitions from abiotic geochemistry to chemoautotrophic prokaryotes, and from prokaryotes to nucleated cells. Philosophical Transactions of the Royal Society of London B: Biological Sciences 358, 59-83 (2003).
3. Russell, M.J. & Martin, W. The rocky roots of the acetyl-CoA pathway. Trends in Biochemical Sciences 29, 358-363 (2004).
4. Martin, W. & Russell, M.J. On the origin of biochemistry at an alkaline hydrothermal vent. Philosophical Transactions of the Royal Society of London B: Biological Sciences 362, 1887-1925 (2007).
5. Martin, W., Baross, J., Kelley, D., & Russell, M.J. Hydrothermal vents and the origin of life. Nature Reviews Microbiology 6, 805-814 (2008).
6. Corliss, J.B., et al. Submarine thermal springs on the Galápagos rift. Science 203, 1073-1083 (1979).
7. Miller, S.L. & Bada, J.L. Submarine hot springs and the origin of life. Nature 334, 609-611 (1988).
8. Russell, M.J., Hall, A.J., & Turner, D. In vitro growth of iron sulfide chimneys: possible culture chambers for origin‐of‐life experiments. Terra Nova 1, 238-241 (1989).
9. Earthguide: Mid-Atlantic Ridge, journal entry, Dec. 12, 2000.
10. Russell, M.J., Nitschke, W., & Branscomb, E. The inevitable journey to being. Philosophical Transactions of the Royal Society of London B: Biological Sciences 368 (2013). Retrieved from doi:10.1098/rstb.2012.0254
11. Mac McClellan, J. What Is the Most Fuel Efficient Airplane? Flyingmag.com (2008).