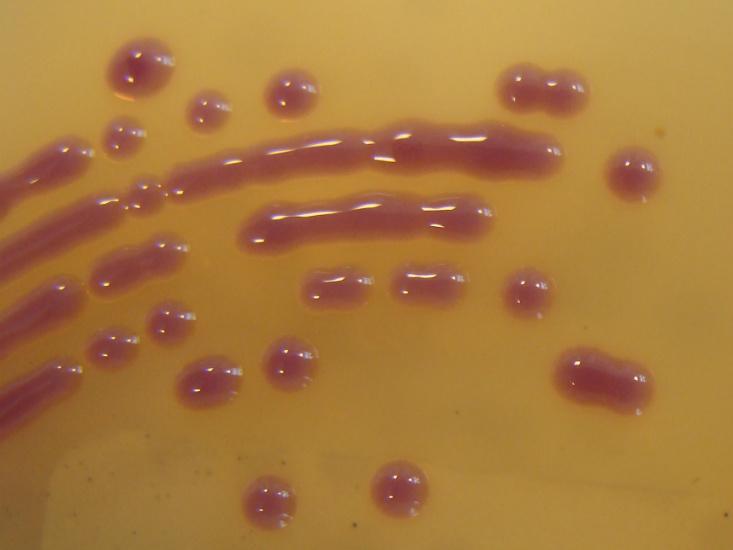
After the attacks of September 11, 2001, and the subsequent anthrax mailings, the United States government started taking the possibility of biological terrorism very seriously. It spent billions of dollars upgrading laboratories to securely study dread diseases, funded new research on how evildoers might weaponize pathogens, and generally turned the nation’s biological-threat indicator to yellow.
Yet there was a terrible irony to this. Even as we looked to our enemies for infectious threats, we—as a society, as a civilization—were busy creating the threats ourselves. The profligate use of antibiotics, both to treat diseases in humans and to spur the growth of factory-farmed animals, has inundated our environments with antibiotics. From an evolutionary perspective, this amounts to massive, constant pressure for microbes to find and share ways to survive the antibiotic onslaught. We’ve created a world that favors, that actively encourages, the emergence of deadlier diseases1.
Over the last few years, as public health experts and crusading journalists sounded the alarms about the dwindling effectiveness of front-line antibiotics, people have become more aware of the problem. The annual toll of drug-resistant bacteria has risen to 23,000 deaths and two million infections in the U.S. alone, and their trajectory is going sharply up; just few decades ago, those numbers were near zero. As bad and scary as the situation seems, many people still don’t realize just how bad and scary it could get.
This fact is neatly embodied by NDM-1, sometimes referred to as the NDM-1 superbug after its discovery in 2008. That’s not quite right—NDM-1 isn’t a specific bug, but rather an enzyme that neutralizes nearly every so-called beta-lactam antibiotics, a class that includes roughly two-thirds of all antibiotics. This it accomplishes with extraordinary alacrity and versatility: The enzyme’s physical geometry is ideal for intercepting beta-lactam antibiotic molecules, and it uses a wide variety of free-floating metals to fuel chemical reactions that break those molecules down2.
In short, NDM-1 is a lean, mean antibiotic-destroying machine—and that’s just the start of the problem. The NDM-1 gene that codes for the enzyme isn’t bound to a nice, predictable location on bacterial chromosomes. Instead it’s found on a stretch of DNA called a transposon, or “jumping gene,” which has the tendency to drift between cells. These can hook up to chromosomes or to structures called plasmids: free-floating, self-replicating pieces of DNA that can contain multiple genes and other pieces of genetic machinery.
Even as we looked to our enemies for infectious threats, we—as a society, as a civilization—were busy creating the threats ourselves.
The NDM-1 gene, it turns out, is frequently found on plasmids, accompanied by a host of other resistance-enabling features. Transposons and plasmids allow bacteria to easily exchange genetic capabilities, and thus adapt to environmental pressures, including antibiotics. They’ve helped create a portable drug-resistance toolbox, readily acquired by most any microbe.
NDM-1 has now been found in at least 15 pathogens, including E. coli and Acinetobacter and the bug that causes pneumonia. (It’s also evolving: There’s also NDM-2 and NDM-3 and so on down to NDM-9, each signifying some new mutation with as-yet-unknown effects.) Travelers have carried NDM-1 to more than 40 countries in Asia, Europe, and the Americas; a team of French physicians called this “a wide and uncontrollable spread of pandemic clones for which new and effective antibiotics are currently not available.” In conjunction with the rise of other drug-resistant bugs, humanity is now confronting the end of a golden age of antibiotics.
Here it’s worth taking a moment to spell out NDM-1’s full name: New Delhi metallo-beta-lactamase 1. It’s named for the locale of its original identification, a fact that angered India’s government, which considered it stigmatizing. It’s certainly easy to imagine NDM-1 evolving there—antibiotic overuse, poor sanitation, and high disease rates have created a perfect storm for drug resistance—but it could just have easily have emerged in the drug-suffused sewage lagoons of some Midwestern U.S. pig farm.
After all, NDM-1’s original genetic components are widespread in the bacterial world. Antibiotic overuse merely brought them together and refined them. And even if new drugs are found to evade NDM-1, they’ll only be a stopgap measure; there are many more molecular mechanisms of drug resistance, and endless incentives for them to coalesce in an antibiotic-flooded world. Unless humanity changes how it uses these drugs, this will almost certainly happen, again and again and again. NDM-1 could be just the first in a series.
“We’re only a couple steps away from any number of other broad-spectrum resistance genes from alternate mechanisms,” says Dennis Livesay, a University of North Carolina at Charlotte molecular biologist and antibiotic resistance specialist. “We are simultaneously on the precipice of several different cliffs.”
(1) What’s both extraordinary and little-appreciated about this is how antibiotic resistance typically carries what scientists call a high fitness cost. As Mark Toleman, a Cardiff University microbiologist who helped discover NDM-1, described in a recent Journal of Infection and Public Health Policy article, drug-disabling mechanisms require a heavy investment of cellular resources; drug-resistant bugs typically reproduce less and spread less easily than non-resistant bugs. In short, the evolutionary deck is stacked against them — but humans have so inundated the world with antibiotics that it doesn’t matter.
(2) Many thanks to Andrzej Joachimiak, a structural biologist at Argonne National Laborator who helped explain how NDM-1 works at the molecular level. Joachimiak is working to design compounds that target NDM-1. It’s a crucially important task, though one that—in the absence of a fundamental change in how societies use antibiotics—will be obviated by the next, inevitable antibiotic-disabling mechanism to evolve and spread in bacteria. “We select for these strains,” said Joachimiak.
Brandon Keim (@9brandon) is a freelance journalist specializing in science, environment, and culture.