TThere were no eyes to see it, but the sun was dimmer in the sky, shining its weak rays on the ground below. The planet was covered in a thick methane atmosphere. The sea had a shiny green color, and where rocks met water, minerals leaked out. Estuaries, creeks, and mud flats were full of tiny life—single-celled bacteria and archaea. This wasn’t life when it first appeared on Earth. This was life on Earth when it was a billion years old.
Life was thriving in the areas where water met air and air met rock. These organisms created a living with metabolisms that got energy from minerals and gases.1 But life was tough. Sulfur and nitrogen, which were the most valuable elements at that time, were scarce and were released slowly from rocks due to weathering. Hydrogen gas, another valuable resource, was consumed quickly and turned into more methane.
Then came a rebel, a type of bacteria that found a way to live on water and sunlight alone.
Outwardly, nothing made the mutineer stand out among other single-celled microbes of its time, except its blue-green color, which eventually gave it the name cyanobacteria. But internally, cyanobacteria produced more energy than any other lifeform before, using the abundant resources of sunlight and water. This new chemical process released a gas that would change the course of life on Earth forever: oxygen.
Tiny cells, preserved for 14 percent of the entire universe’s lifespan, have been waiting.
Today, oxygen is associated with life, but to most organisms living on young Earth, it was deadly. Oxygen is highly reactive—it removes electrons from metals and attaches itself to elements like nitrogen and sulfur, breaking the stable molecules that most microbes relied on for survival. Unintentionally, the new arrival was making the little available food for microbes inedible. When not reacting with other elements, O is so aggressive that it even reacts with itself, releasing free radicals. These molecule fragments are so unstable that they attack most chemical bonds randomly, causing biological chaos. Exposed to high levels of these radicals, all living cells quickly deteriorate. It’s amazing that cyanobacteria survived their own gas. Essentially, they were setting themselves on fire.
The first cyanobacteria may have tried producing oxygen-based energy only when they had no other fuel left—a last resort to be used in desperate times until they gradually developed the ability to detoxify the oxygen. With the planet's abundance of water and light, cyanobacteria stumbled upon an untapped source of energy. Once they found a way to oxidize water without self-destruction, a revolution began.
That revolution was photosynthesis, using power from light (“phos” in ancient Greek). Cyanobacteria gathered the sun’s rays and used their energy to split water particles. Breaking water releases a powerful burst of energy, which the cyanobacteria turned into energy currency, a sugar to store and consume when necessary. The opportunities photosynthesis offered were too many compared to the drawbacks; there was no way to reverse the situation.
The discovery of photosynthesis also allowed other tiny organisms to benefit from the energy captured by cyanobacteria from the sun. Without this important process, there wouldn't have been enough energy in the food chain for more complex life forms like moss, millipedes, house cats, and humans. Modern cyanobacteria and other light-absorbing organisms continue to thrive and produce the oxygen we breathe.
PCreating a narrative about organisms that existed 3 billion years ago, which were too tiny to see and lived in a very different environment, feels like creating a myth. We know only a small fraction of the current microbes on Earth. How can we create a story about the origin of one that lived so long ago?
For a long time, fossils have been the best evidence we had for ancient organisms. New technology has made the search for these fossils go from large dinosaur bones to very small microfossils.2 . In her laboratory in Belgium, biologist Emmanuelle Javaux uses electron microscopes and chemical tracing to look for ancient and seemingly invisible beings. The oldest cyanobacteria found in the fossil record date back to the Proterozoic era, about 1.9 billion years ago. The discovery is mind-boggling: tiny cells, just 10 microns long, preserved for 14% of the entire universe's existence. They were found in a layer at the top of a microbial mat that had turned into dense crystalline rock. It was clearly a cyanobacterium. "And the way they divide is very similar to some modern cyanobacteria," Javaux says. a more recent find
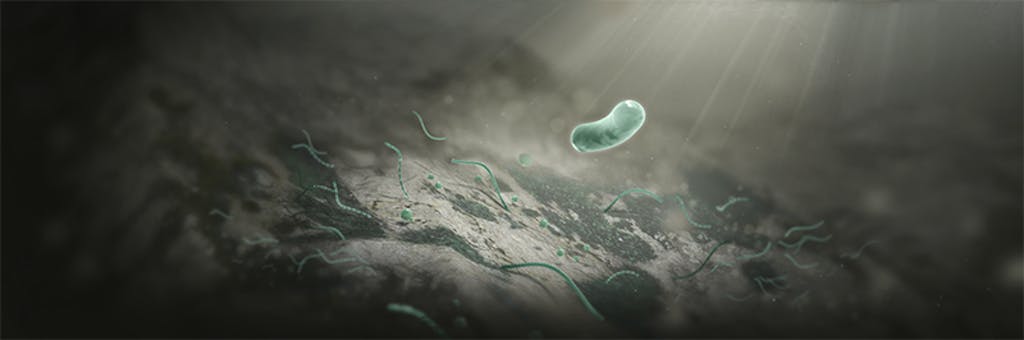
In In a more recent discovery, Javaux has studied cyanobacterial microfossils to uncover complex structures within their cells—small membranes that provide the first "direct evidence of a cell performing oxygenic photosynthesis," she says.The tiny cells in question are 1.75 billion years old. Further chemical analysis can provide insights into the early cyanobacteria's metabolism and biology. For Javaux, "I am always amazed to find such old cells and look at them under the microscope … they are treasures." However, even these incredibly ancient microfossils are about 1 billion years too young to create a complete origin story of the pioneering3 creators of photosynthesis Without ancestral fossils, other scientists are examining the genomes of modern microbes, their living descendants. "Because we're using genes and phylogenies, we can work backward in time earlier than the fossil record," says Greg Fournier, professor of geobiology at MIT. Identifying pieces of microbial genomes that are the oldest becomes scientists' first step in building a partial profile of the original rebel..4
But tracing the evolutionary path back using only modern cyanobacteria is also challenging. "We don't have direct ancestors," Fournier says. "We have this big, long branch in our tree that separates cyanobacteria from everything else. Along that branch, all of the evolution of oxygenic photosynthesis must have occurred," but the stepping-stones are unknown. "Maybe they're out there … but we haven't found them yet," Fournier says. "It's a little bit like trying to understand something about human evolution without any knowledge of the great apes," adds Woodward Fischer, professor of geobiology at Caltech.
Another interesting way to understand the history of these planet-changers is to examine the environmental impact of their breath. Because oxygen is reactive and can cause iron to rust, large-scale oxygen emissions leave a noticeable mark in the rock record. A significant turning point in our planet's history is the "Great Oxidation Event" (GOE) around 2.4 billion years ago. This is when oxygen levels first reached 1 to 5 percent of the atmosphere's composition. Scientists believe sustained cyanobacterial activity could have led to this dramatic increase in the planet's atmosphere.
dramatic buildup in the planet's atmosphere. But the first
Today, oxygen feels synonymous with living, but to most organisms then, it was lethal.
hints of oxygenbefore the GOE are hard to identify.To look for traces, some scientists are examining ancient rocks. One team of scientists, including Timothy Lyons, a professor at the University of California, Riverside, claims to have detected small5 bursts of oxygenin the atmosphere, in specific areas, about 50 million years before the GOE. These may be the remains of cyanobacterial activity or have non-biological causes, such as geological processes releasing oxygen from deformed minerals.ago in the run-up to the GOE. These could be remnants of cyanobacterial activity, as suggested by Fournier, or they might have non-biological origins. There are specific prior geological situations where minerals are deformed under pressure and react with water, releasing oxygen. These may be different aspects of the same story: Cyanobacteria first evolved 3 billion years ago, but it took them—probably with help from geological processes—another 600 million years to change the planet. “You can divide Earth history in about half,” Fischer says, “there’s a half without oxygen and a half with oxygen,” before and after the GOE—before and after cyanobacteria took off and revolutionized the environment.6
Although it is amazing how cyanobacteria survived the toxic impact of oxygen, the overall effect of accumulated oxygen on the climate was catastrophic. Some scientists refer to the GOE as the “Great Oxygen Catastrophe.” It not only poisoned the air, but it also removed the atmosphere's methane layer, leading to a prolonged icy period. The Earth became covered in ice for 85 million years, and the surviving organisms may have led a lifestyle similar to
Tthe microbes in today's polar ice . The persistent oxygen levels, lack of nutrients, and cold temperatures would have made most areas uninhabitable for microbes that had thrived for 1 billion years. All of this resulted from a tiny chemical change.oxygen's dominating presence, scarce nutrients, and frigid temperatures would have made most regions where microbes lived for a billion years completely uninhabitable. All thanks to a single spark, a microbial chemical trickery. With oxygen becoming dominant, all previous life forms on Earth—obligate anaerobes (bacteria and archaea that cannot tolerate even small amounts of O.
They were forced into refuge in environments without oxygen. They still exist in such refugia today, in stagnant pools, mud pots—like the bubbling acid hot springs at Yellowstone National Park—or near hydrothermal vents deep in the ocean, pockets of the planet that retain echoes of what most of it once was like.
Other organisms became creative, incorporating the newly abundant compound for their own benefit. One offshoot was respiration, the metabolic process by which most cells that live exposed to oxygen break down sugars to release energy. Respiration is an ancient metabolic invention andprobably developed soon after photosynthesis
Temperatures plummeted so low it turned the Earth into a giant “snowball.”
. However, respiration cannot keep up with photosynthesis; O must be constantly produced to remain a dominant force in the atmosphere.The solitary, rebellious innovation of exploding water to release energy has not been replicated in the 3 billion years of continuous evolution, since cyanobacteria first appeared. Instead, it has been passed along the tree of life like a torch of Promethean flame. The vegetation that covers our Earth, coloring our landscapes green, relies on this ancient alchemy. Rather than inventing their own version, roughly 1.5 billion years ago a bacterium
engulfed a cyanobacterium
and integrated its metabolisms completely into its own cellular blueprints—resulting in the emergence of an algal cell. This next-level innovation, the enslavement of cyanobacteria, was so profound that oxygen concentrations rose exponentially as algae spread across the ocean. It is estimated that today algae produce roughly half of the oxygen on the planet. Fast-forward another billion years, and a portion of these algae gradually evolved into land plants, passing the knowledge of cyanobacterial photosynthesis—nested within its cells—upward along the tree of life. The cyanobacteria’s innovation has both the simplicity and depth of a creation story. On the surface, all it provides is the combination of sunlight and water to create fuel—and release oxygen. However, scientists are still puzzling over the inner workings of today’s cyanobacteria. “We still don’t know all the tricks that allow them to do this so easily,” Fischer says. “You know, the best, fanciest chemists in the building across the quad from me, right?” he exclaimed excitedly during our conversation, pointing outside his office window in Caltech, “They would just love to be able to do chemistry so elegantly, in the way that it takes place in cyanobacteria, algae, and plants.” A single organism can, unknowingly, alter the atmosphere—and, with it, the entire planet’s trajectory. And although it is difficult to imagine, as we live through a climate catastrophe of our own making driven by high levels of CO
, in the constant, long fluxes of time, there might also be, “a future Earth—millions and billions of years down the line—where CO
might be so low that it becomes limiting for oxygenic photosynthesis,” Lyons suggests. As our aging sun burns even brighter then, it might eliminate all COout of the air, pushing cyanobacteria and the other photosynthesizers to their sunset. “We’re not going to be around to see it—nothing like us is going to be around to see it,” Lyons says, but “the world may return to an anaerobic planet. We may return whence we came.” And the stage will be set for a new rebel. 1. Canfield, D.E., Rosing, M.T., & Bjerrum, C. Early anaerobic metabolisms. Philosophical Transactions of the Royal Society B
References
, 1819-1836 (2006). 2. Demoulin, C.F., 361Cyanobacteria evolution: Insight from the fossil record.
Free Radical Biology and Medicine et al. , 206-223 (2019). 3. Demoulin, C.F., Lara, Y.J., Lambion, A., & Javaux, E.J. Oldest thylakoids in fossil cells directly evidence oxygenic photosynthesis. 140, 529-534 (2024).
4. Fournier, G.P., Nature 625The Archean origin of oxygenic photosynthesis and extant cyanobacterial lineages.
Proceedings of the Royal Society B et al. , 20210675 (2021). 5. Anbar, A.D., 288A whiff of oxygen before the Great Oxidation Event?
, 1903-1906 (2007). et al. A mineral-based origin of Earth’s initial hydrogen peroxide and molecular oxygen. Science 317Proceedings of the National Academy of Sciences
6. He, H., et al. e2221984120 (2023). How cyanobacteria killed one climate and created our habitable Earth. 120, e2221984120 (2023).