Humans age gradually, but some animals do all their aging in a rush at the end of life, while others don’t age at all, and a few can even age backward. The variety of aging patterns in nature should be a caution sign to anyone inclined to generalize—particularly the generalization that aging is inevitable.
Bacteria reproduce symmetrically, just dividing in two. What could “aging” mean for bacteria since, after reproduction, there is no distinction between parent and child? Single-cell protists like the amoeba also reproduce symmetrically, but curiously, they invented a way to age nevertheless. And even among macroscopic life forms, life spans of organisms are immensely variable in a way that is finely tuned to local ecologies and reproduction rates. This can hardly be the result of a universal, inexorable process; in fact, such fine-tuning to circumstance is the signature of an adaptation.
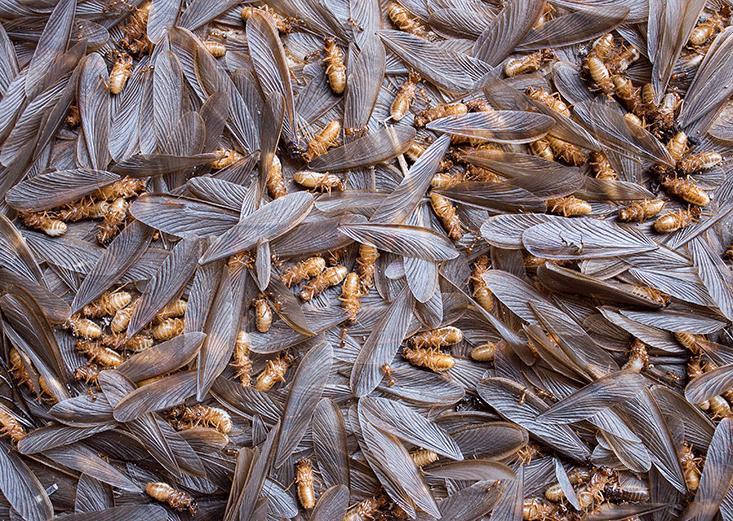
Life spans range from Methuselans great and small to genetic kamikazes that die of a spring afternoon. Submerged dragonflies live four months, adult mayflies half an hour. We live some 70-odd years; but the meristem of the ginkgo may be millions of years old. This range becomes all the more impressive when we realize that the genetic basis for aging is widely shared across different species, from yeast cells on up to whales. Somehow, the same genetic machinery, inherited from our common ancestors at the dawn of life on Earth, has been molded to generate life spans ranging from hours (yeast cells) to thousands of years (sequoia trees and quaking aspen).
And it is not only the length of life but the pattern of deterioration within that time that varies widely. Aging can occur at a steady pace through the course of an entire lifetime (most lizards and birds), or there can be no aging at all for decades at a time, followed by sudden death (cicadas and century plants).
Our own “inner assassin” works with stealth, like an evil empress gradually poisoning her husband; but other species have inner killers that do their deed far more quickly, and still others appear to have no genetic death programs at all. Such variety is a sure signal for a feature molded by active natural selection, not an immutable law of entropy.
As the biomarkers of aging vary widely from one species to the next—indeed, from one individual to the next—it’s difficult to come up with a single universal definition. A man may be prematurely gray, and a naked mole rat baby may be covered with wrinkles. For the actuary, however, the question has a clear answer, even if it’s one only a statistician could love: Aging is an increase in the mortality rate. In other words, as an animal gets older, it suffers an ever-higher risk of death.
For example, a 20-year-old man has 99.9 percent chance of living to see his 21st birthday. This is to say that his chance of dying is 1 in 1,000 per year. If this were to continue, then a 40-year-old would also have a 1 in 1,000 chance of dying before his 41st birthday. We’d call that “no aging.” In reality, a 40-year-old has a 2 in 1,000 chance of dying before his 41st birthday. This doubling of his mortality risk over 20 years is evidence of gradual aging.
It gets worse. The risk for a 60-year-old is 10 in 1,000 and for an 80-year-old, 60 in 1,000.
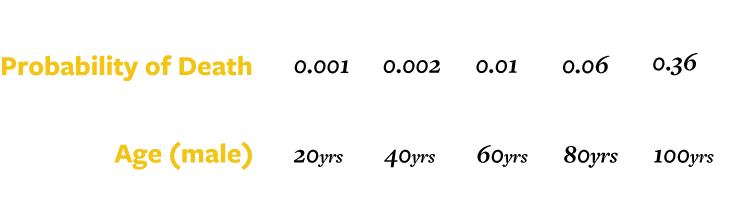
Not only does the risk of dying increase, it increases faster and faster. An increase in the deterioration or the chances of dying with each passing year, such as occurs in us after reaching adulthood, is called “accelerating senescence.” But other species have different patterns. The probability of death may increase and then level out: “decelerating senescence,” or even a “mortality plateau.” If we commit to this definition for aging, we are bound to say that if the probability of death doesn’t increase, then the species doesn’t age at all. It is consistent, if stranger, to say that if the probability of death goes down from one year to the next that a species is aging backward, which is called “negative senescence.”
There’s a second objective measure of aging, and that is decline in fertility. Just as mortality is defined as the probability of death, fertility is defined as the probability of reproduction. Men lose fertility gradually over their adult life. Women lose their fertility more rapidly, and fertility drops to zero at menopause. But different species have different patterns, different schedules. In some species, fertility increases over much of the life span, another form of “negative senescence.”
For example, Blanding’s turtle, a species of box turtle common in the American Midwest, matures slowly over decades, and it doesn’t keep growing, but it does continue to increase in fertility. Apparently, its risk of dying also declines with age. From an evolutionary perspective, the loss of fertility is primary. From the perspective of natural selection, once you’re no longer able to reproduce, you might as well be dead.
Nature can do whatever she wishes with aging (or non-aging). Any time scale is possible, and any shape is possible.
We find it natural to classify different species as living a long or a short time, to lump together the insects that live for a day and distinguish them from the trees and whales that live hundreds of years. But much of that difference can be attributed to size. Everything from growth to reproduction to aging must occur more slowly in a behemoth with a slow metabolism and tons of tissue to nourish. So we are inclined to be more impressed with a honeybee that lives 20 years than we are with a moose that lives 20 years.
But suppose we were to remove length of life completely from consideration and compare different species based on the shape rather than the duration of their life histories. However long or short the life span, we display it in the same size box for comparison. Rather than asking how long they live, ask instead whether their populations tend to die out gradually, or if many die in infancy and fewer later on, or if all the deaths bunch up at the end of the life cycle. A chart published in a paper in Nature in 2014 does just this, and what emerges from this picture is the breadth of nature’s ingenuity. Every conceivable combination is represented, with rapid aging and no aging and backward aging, paired with life spans of weeks or years or centuries. The strange bedfellows that appear as neighbors on the chart are utterly unexpected. For example, at the top of the chart, with low mortality that rises suddenly at the end of life, humans are joined by lab worms and tropical fish (guppies)! In fact, in terms of aging profiles, we humans look more like the lab worm than the chimpanzee.
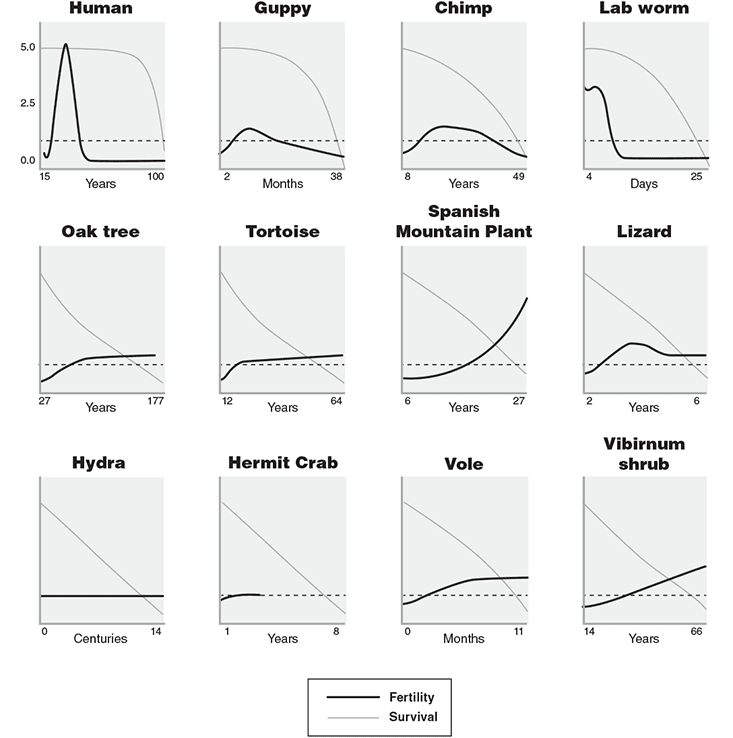
The above graphs show the varieties of ways that animals and plants age in the wild. The light downward line in each frame is the survival curve, and the bold curve underneath is fertility. The downward slope of the survival line just means that fewer and fewer individuals are left alive as time goes on. The way this graph has been constructed, a straight line going diagonally downward is neutral, or no aging at all. Lines that are humped over the diagonal represent normal aging, while lines that curve under the diagonal represent reverse aging, or “negative senescence.” For example, the line for humans stays flat for a long while and then descends sharply; that means that many people are living out a full life span, and then their deaths are all clustered in their 80s and 90s (the stats were recorded for modern-day Japan).
But for animals and plants in the bottom two rows, the death rates are steadier. For turtles and oak trees, in fact, the curves flatten out. That means that there are less of them dying old than dying young, which is aging in reverse.
The bold line, representing fertility, is straightforward. Fertility may rise as an animal or plant grows bigger, or it may fall with reproductive aging—for example, in menopause. Notice that animals in the top row lose all their fertility well before they are dead. This poses an evolutionary conundrum in its own right.1
In this diagram, if the survival curve is a straight diagonal, that corresponds to no aging—for example, the hydra and the hermit crab. (The hydra is like a freshwater jellyfish, a quarter inch long and found in ponds.) All the animals in the top row show “true aging”—they are more likely to die as they get older. The next two rows show plants and animals that don’t age or that age in reverse. For the latter case, the older they are, the less the risk of death. Most trees are like this, and tortoises follow the same pattern, as do clams and sharks (not pictured).
The lower, bold curve in each frame is fertility. Animals in the top row stop reproducing well before they are likely to die. This poses an evolutionary conundrum for neo-Darwinist orthodoxy—if the sole target of natural selection is to maximize reproduction, then why has evolution allowed reproduction to fall to zero while so many remain alive? The rising fertility curves indicate increased reproduction with age, which is another kind of negative senescence. When you think about a tree that grows larger with each passing year, it’s not so surprising that it’s making more seeds the older it gets. The Spanish mountain plant in the third row is Borderea pyrenaica, a plant that grows out on the rocky cliffs of the Pyrenees mountains. If undisturbed, it can live to 300 years or more with no sign of aging; but notice that its fertility doesn’t really get going until it is more than 20 years old.
The message of this diagram is nature can do whatever she wishes with aging (or non-aging). Any time scale is possible, and any shape is possible, and each species is exquisitely adapted to its ecological circumstance. There are no constraints.
Instant Aging
Aging to death can be rapid and sudden at the end of a reproductive cycle. Sudden post-reproductive death is common in nature, affecting organisms as varied as mayflies, octopuses, and salmon, not to mention thousands of annual flowering plants. Biologists refer to this life story as “semelparity” (from Latin “single birth”).
The cause of death in semelparous organisms varies widely. Octopuses just stop eating. Praying mantis males make an ultimate reproductive sacrifice, giving themselves up as snacks to their female partners. Salmon destroy their own bodies with a blaze of steroids.
By the time the adult salmon reach their spawning ground, their metabolisms are in terminal collapse. Their adrenal glands are pumping out steroids (glucocorticoids) that cause accelerated—almost instant—aging. They’ve stopped eating. Moreover, the steroids have caused their immune systems to collapse, so their bodies are covered with fungal infections. Kidneys atrophy, while the adjacent cells (called interregnal cells, associated with the steroids) become greatly enlarged. The circulatory systems of the rapidly deteriorating fish are also affected. Their arteries develop lesions that, interestingly, appear akin to those responsible for heart disease in aging humans. The swim upstream is arduous, but it is not the mechanical beating that fatally damages their bodies. It is rather a cascade of nasty biochemical changes, genetically timed to follow on the heels of spawning.
Some organisms are genetically programmed not to eat after reproduction and starve as a result; it’s quicker and surer than traditional aging. Mayflies entering adulthood have no mouth or digestive system whatever. Elephants chomp and grind so many stalks and leaves during a lifetime that they wear out six full sets of teeth. But when the sixth set is gone, they won’t grow another, and the pachyderms starve to death.
Queen bees show no symptoms of senescence. They are ageless wonders.
Longevity
In 2014, photographer Rachel Sussman published a coffee-table volume of her ancient subjects entitled, The Oldest Living Things in the World. All of them are plants. One reason for this, at least in comparison to ambulatory animals, is that plants don’t have to worry about leg muscles strong enough to walk. Confined to one location, they can grow larger and sturdier, older and far more fertile than any animal, and reap the benefits of seniority.
Plants have another longevity secret. Early in the life of a developing animal, the sex cells, or germ line, segregate from the rest of the body, or soma. Only the germ line must be preserved immaculate to become the next generation; the body can afford to be sloppier with cells of the soma and take shortcuts as they reproduce themselves. But plants have a different system. The germ line and soma never really segregate. Plants, like animals, have stem cells, and in plants those stem cells give rise not only to new plant growth but also to the seeds and pollen that is destined to become the next generation. In a tree, the stem cells are located in a thin layer under the bark, called the meristem. The meristem extends into every branch and twig of the tree and gives rise to new leaves and also to buds and seeds. In some ginkgoes, nonflowering trees that date back to the Permian 270 million years ago, this meristem layer may be millions of years old.
Still, it seems that most trees have a characteristic age, after which death finally becomes more likely with each passing year. Shoots (“epicormic sprouts”) begin to grow directly from the tree trunk as growth at the outermost branches slows. There is some indication that trees become more vulnerable to fungus and disease with old age, but for the most part, old trees succumb to the mechanical hazards of excess size. The very ability to continue growing that offers them the possibility of “reverse aging” over so many decades proves in the end to be their downfall.
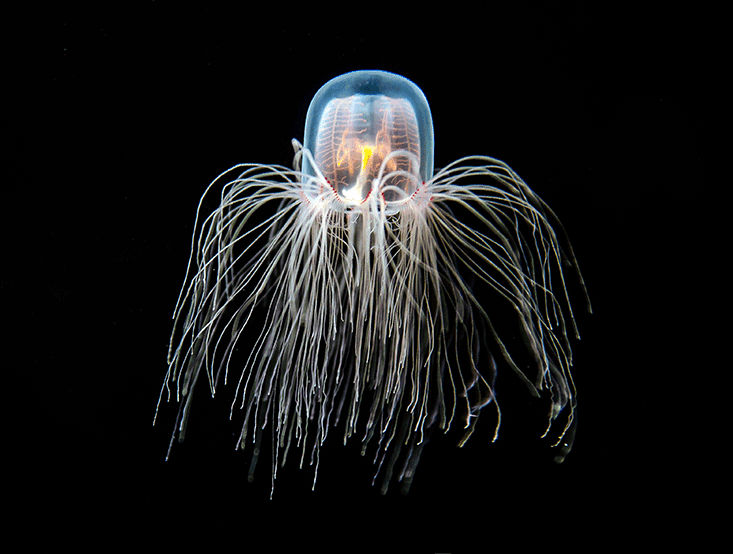
Aging in Reverse
Though it doesn’t enroll in kindergarten at age 65, the immortal medusoid Turritopsis nutricula achieved its 15 minutes of fame when it was hailed as “the immortal jellyfish” in science news articles of 2010. The adult Turritopsis has inherited a neat trick: After spawning its polyps, it regresses back to a polyp, beginning its life anew. This is accomplished by turning adult cells back into stem cells, going against the usual developmental direction from stem cells to differentiated cells—in essence driving backward down a one-way developmental street. Headlines called Turritopsis the “Benjamin Button of the Sea.”
Dermestid beetles (Trogoderma glabrum) perform a similar trick, but only when starved. As they play life out on a carcass in the woods, the beetles go through six different larval stages in succession, looking like a grub, and then a millipede, and then a water glider before ending up as a six-legged beetle. A pair of entomologists working at the University of Wisconsin in 1972 isolated the sixth-stage larvae (when they were just ready to become adults) in test tubes and discovered that without food, they regressed to stage-five larvae. If they were deprived of food for many days, they would actually shrink and regress backward through the stages until they looked like newly hatched maggots. Then, if feeding was resumed, they would go forward again through the developmental stages and become adults with normal life spans. They found they were able to repeat the cycle over and over again, allowing them to grow to stage six and then starving them back down to stage one, thereby extending their life spans from eight weeks to more than two years.
Ancient Aging
Hydras are radially symmetrical invertebrates, each with a mouth on a stalk, surrounded by tentacles, which grow back when cut off—like the many-headed monster of Greek mythology for which they are named. They have been studied for four years at a time, starting with specimens of various ages collected in the wild, and they don’t seem to die on their own or to become more vulnerable to predators or disease. In the human body, certain cells, such as blood cells, skin, and those of the stomach lining, slough off and regenerate continuously. The hydra’s whole body is like this, regenerating itself from stem cell bedrock every few days. Some cells slough off and die; others, when large enough, grow into hydra clones that bud from the stalk-body to strike out on their own. This is an ancient style of reproduction, making do without sex. For the hydra, sex is optional—an occasional indulgence.
One recent article claims that the hydra does indeed grow older, and it shows it by slowing its rate of cloning. The author suggests that perhaps clones inherit their parents’ age. The hypothesis is that only sexual reproduction resets the aging clock. If this is true, then the hydra’s style of aging is a throwback to protists, ancestral microbes more complex than bacteria—some of which have a limited life span, being able to divide only so many times until they run out of reproductive gas—unless they are jumpstarted by exchanging genes (a kind of protist version of sex), which resets their aging clock. Amoebas and microbes of the genus Paramecium are examples of these protists, single cells in a vast lineage that has anciently radiated into over 100,000 species and includes all the seaweeds, slime molds, and ciliates and other organisms that do not belong to the animal, fungal, plant, or bacteria kingdoms.
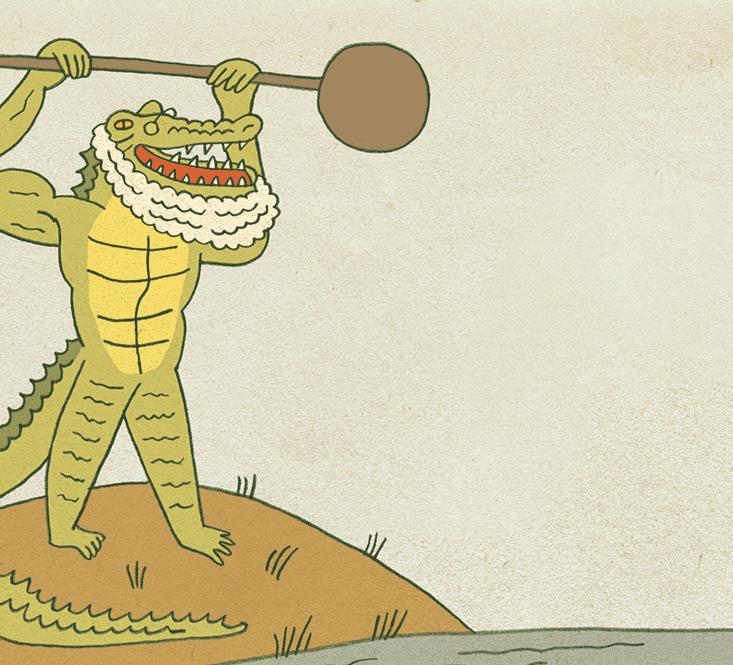
Turning Aging Off
Queen bees and worker bees have the same genes but very different life spans. In the case of the queen bee, royal jelly switches off aging. When a new hive begins, nurse bees select—arbitrarily so far as we can tell—one larva to be feted with the liquid diet of royalty. Some physiologically active chemical ambrosia in the royal jelly triggers the lucky bee to grow into a queen instead of a worker. The royal jelly confers upon the queen the overdeveloped gonads that give her a distinctive size and shape. The queen makes one flight at the beginning of her career, during which she might mate with a dozen different drones, storing their sperm for years to come.
Weighted down with eggs, the full-grown queen, too heavy to fly, becomes a reproducing machine: she lays at a prodigious rate of about 2,000 per day, more than her entire body weight. Of course, such reproductive regality requires a suite of specialized workers to feed her, remove her waste, and transmit her pheromones (chemical signals) to the rest of the hive.
Worker bees live but a few weeks and then die of old age. And they don’t just wear out from broken body parts, the rough-and-tumble worlds through which they fly. We know this because their survival follows a familiar mathematical form, called the gompertz curve, the characteristic tailing of survival, typical for humans and many other animals, indicating aging. Meanwhile, queen bees, though their genes are identical to those of the workers, show no symptoms of senescence. They can live and lay for years and sometimes, if the hive is healthy and stable, for decades. They are ageless wonders. The queen dies after running out of the sperm she received during her nuptial flight. At that point, she may continue to lay eggs, but they come out unfertilized and can only grow into stingless drones. Then, the same workers that formerly attended her assassinate the depleted queen. They swarm about her, stinging her to death.
Post-Reproductive Life Spans
Why is there a menopause? We care for our young and our extended families, and our devotion continues after our children have grown and become parents themselves. Hence, the standard explanation for life that continues after fertility ends is called the “grandmother hypothesis.” Women have a genetic interest in seeing their grandchildren grow up healthy. Maybe at age 60 they can contribute more to their own genetic legacy by caring for their grandchildren than by having more babies of their own. This is a hypothesis that sounds reasonable, at least for humans, but a number of demographic researchers have found that when they do the numbers, it’s hard to make it work.
Whales and elephants are also examples of organisms who outlive their fertility. They are social animals, too. Perhaps they are more important to their grandchildren than we know. But in the chart, there are other animals that go right on living after their fertility has ended. These include guppies, water fleas, roundworms, and bdelloid rotifers, all of which make deadbeat dads look like Mary Poppins. All these animals lay eggs, and that’s it. None of them lifts a wing or a fin to care for their young, let alone their grandchildren. Yet modern evolutionary theory says that there is no natural selection to keep them alive, and thus we should expect them to be kaput.
In 2011, Charles Goodnight and I had an idea about how post-reproductive life span might evolve, an idea that sounds pretty unlikely in the abstract, but when we did the numbers, it actually panned out. An older, “retired” segment of the population, we argued, serves to keep the population stable over cycles of feast and famine. When times are good, they eat the excess food and help prevent population overshoot. When food is scarce, they are the first to die.
Styles of aging in nature are just about as diverse as they can be, which suggests that nature is able to turn aging on and off at will. With this in mind, we may be forgiven for regarding theories that explain why aging must exist with extreme skepticism. Whatever our theory of aging turns out to be, it had better make room for plasticity, diversity, and exceptions.
Theoretical-biologist Josh Mitteldorf has a Ph.D. from the University of Pennsylvania. He runs the website AgingAdvice.org, and writes a weekly column for ScienceBlog.com. Mitteldorf has had visiting research and teaching positions at various universities including MIT, Harvard, and Berkeley.
Dorion Sagan is a celebrated writer, ecological philosopher, and theorist. His essays, articles, and book reviews have appeared in Natural History, Smithsonian, Wired, New Scientist, and The New York Times, among others.
Reference
1. Jones, O.R., et al., Diversity of ageing across the tree of life. Nature 505, 169–173 (2014).
Excerpted from Cracking the Aging Code by Josh Mitteldorf and Dorion Sagan. Copyright © by the authors and reprinted by permission of Flatiron Books, a division of Holtzbrinck Publishers Ltd.