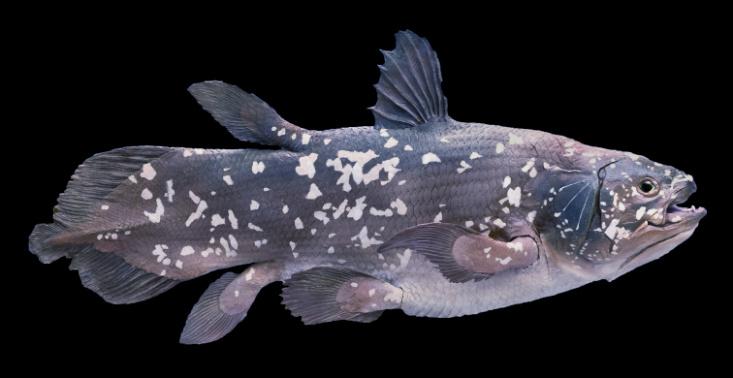
It was an image in a book of a sparkly blue fish—a West Indian Ocean coelacanth—that inspired German painter Franziska Schenk to begin a project that would occupy much of her adult life. “It was mysterious and beautiful,” she says, “and as a child I had been enamored with the sea.” She became engrossed with trying to capture that elusive beauty in her art. Making iridescent paintings would not only prove a technical challenge, but would also force Schenk to explore some of the exotic ways that nature creates color.
Her first attempts were giant portraits of menacing fish called grouper. But efforts to include a golden, shining background failed due to the yellow paints she tried—they were “dull,” she wrote later. “The vivid golden and more familiar silver beams of iridescence generated by many fish, on the other hand, appear identical to the actual precious metal.” She couldn’t find any paint that would give the same shimmery look that fascinated her. “I was frustrated,” she recalls. “I wanted to use the same technology as the fish.”
It took a year of experimentation to understand how to turn the powder into a spreadable paint and mix it without destroying its optical qualities.
For centuries, scientists have sought to unlock the secrets of so-called structural color, the source of nature’s most fantastic hues. In 1730, building on his new theory of colors, Sir Isaac Newton hypothesized that peacock feathers shimmer by physically splitting light like a prism, which was on the right track but incomplete. At the turn of the 19th century, physicist Thomas Young finally figured out that the wave nature of light could explain how thin transparent films create iridescence. Pigments, the common type of coloring found in almost everything around us, create color by absorbing certain wavelengths of light, or colors, and reflecting others; when the reflected ones bounce to our eyes, we perceive them as colors. Structural colors, like the ones you can see on a soap bubble, work entirely differently. When light strikes a thin film like a soap bubble, some of the light is reflected upward by the top surface of the film. But some of the light continues through the film, striking the bottom edge and getting reflected back upward. The beams reflected from the top and from the bottom then combine, Young realized, in accordance with his new understanding of light acting as waves. In that combination, some wavelengths of light get amplified, while some get diminished, depending on the chemical nature of the film and its thickness. The colors that get amplified are the intense colors we see. The way the waves combine also depends on the angle from which our eyes view that light, which explains why iridescent colors shimmer and change when viewed from different angles.
That phenomenon explains the rainbows seen on oil films, bubbles, and many bugs, as Schenk learned in a series of residencies with scientists. At a British aquarium in 2004, she studied the squid-like cuttlefish, which uses skin elements called chromatophores, some of them iridescent, to mimic myriad colors and blend in to its surroundings. A residency at the Natural History Museum in London three years later allowed her to observe pinned iridescent Morpho butterflies. Their wings derive their deep azure hue from stacked layers of a transparent structure called chitin. And during recent visits to a Dutch lab she learned how to measure the optical properties of her work.
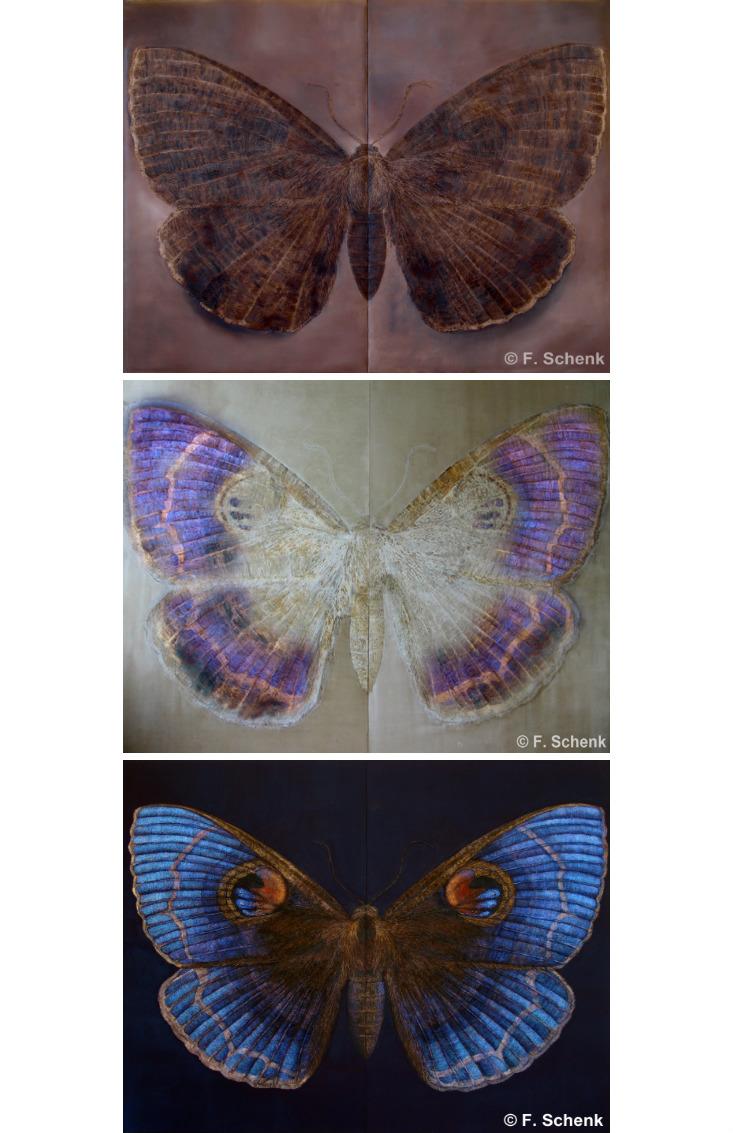
Meanwhile Schenk endeavored to create iridescent paints that could be applied to canvas. Commercially available paints billed as iridescent showed only pale, faintly metallic colors, not nature’s vividly shimmering hues. Then she obtained top-of-the-line iridescent materials that were supposed to display shimmering purple, gold, and red. Drab grey powders, they were unlike any pigment she had seen before. They consisted of minuscule flakes made of silica coated with several metal-oxide layers. It took a year of experimentation to understand how to turn the powder into a spreadable paint and mix it without destroying its optical qualities.
Then she had to learn how to blend her iridescent paints into a palette of colors. “It was counterintuitive,” she says. “With traditional pigmentary colors, you mix a yellow and a blue and you get a green.” The paints she’d created, however, use so-called additive color rules, which describe what happens when you mix light, rather than pigment. “If you mix a gold and a metallic blue you get white … I had to forget all I had learned in color theory.”
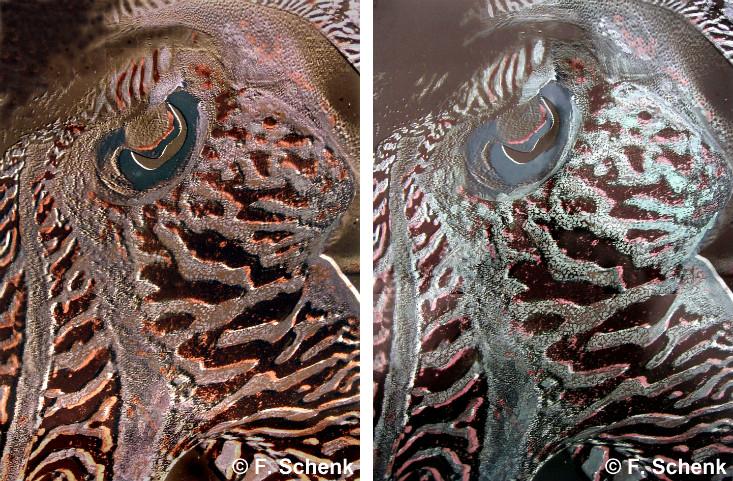
Borrowing nature’s color tricks came next. Magenta spots on wings of butterflies in the Colotis genus, for example, combine a red background pigment with layered reflectors that give a blue sheen. “My paintings became layered and built the way the butterfly wings were,” says Schenk. In 2013, she reproduced the orange underside of the Japanese jewel beetle, its green top shell, and its violet stripes, and proved their likeness to the real thing by comparing reflectance measurements.
Schenk has exhibited her paintings in Germany, the United Kingdom, and soon, the Netherlands. The works have a realism one would expect in a photograph, but then they shimmer or flash, capturing the dynamic nature of an iridescent specimen in a way a conventional image cannot. They change their rich colors as the viewer moves, like the butterfly and beetle tissues they depict. “It’s almost like a competition between the photograph and the painting,” says Schenk. “For the moment, I’m winning.”
Eli Kintisch is a correspondent for Science magazine. His 2010 book, Hack the Planet, explored geoengineering, the deliberate tinkering with the atmosphere to counteract global warming.
This article originally appeared on our blog, Facts So Romantic, in April 2014.